Nanodiscs are artificial scaffolds that mimic the phospholipid bilayer surrounding every living cell. They allow the reconstitution and stabilization of membrane proteins (MPs) in a native conformation, thus enabling functional studies of these important drug targets. Nanodiscs might also pave the way for the application of membrane proteins in future diagnostic assays.
What are Nanodiscs?
Nanodisc proteins have gained significant interest in recent years due to their ability to mimic the natural lipid environment and provide a stable platform for membrane proteins. Membrane scaffold protein (MSP) Nanodiscs, as shown in figure 1, consists of a synthetic bilayer of phospholipids (red) that are held together by a ring of MSPs (blue) allowing the reconstitution of membrane proteins (dark blue).
In the nanodisc, the synthetic phospholipid bilayer of phospholipid is held together by a ring formed by two molecules of an amphipathic helical membrane scaffold protein (MSP) derived from apolipoprotein A-I of human, rat, or mouse origin (Fig.1). Since the lipophilic components of the membrane proteins are masked inside the lipid bilayer, the membrane proteins can be purified via standard chromatography methods without the addition of detergent.
For purification, the membrane protein itself can be tagged, or purification can be performed utilizing the His-tag fused to the MSP. Membrane protein-nanodisc complexes show an increased stability compared to detergent-solubilized membrane proteins.
Why use Nanodiscs?
Nanodiscs are not the only option to study correctly folded, active membrane proteins. However, they have a number of advantages compared to commonly used methods:
Detergent
- + keep membrane proteins in solution
- + diversity of detergents
- - Possible negative effect on membrane protein stability and/or activity – needs optimization
- - Pronounced effect of membrane curvature
- - Potential interference with many assays – need optimization
- - Concentration difficult to determine
- - Optical artifacts (absorbance, light scattering)
Liposome
- + Phospholipid bilayer
- + Provides compartmentalization (e.g. for ion channels)
- - Incompatible with crystallization; difficult in NMR
- - Large and unstable structure
- - Difficult to prepare (defined size, stoichiometry)
Nanodisc
- + Self-assembly in native-like lipid environment
- + No membrane curvature
- + Water-soluble
- + flexible phospholipid choice / maintenance of original membrane lipid composition
- + variable, defined size (MSP variants)
- + suitable for a wide range of biophysical and biochemical studies, including NMR and SPR
- + measurement of interactions on both sides of the membrane
- - no compartimentalization, measurement of ion channel activity difficult
Available MSP Nanodisc Variants
antibodies-online offers following MSP Nanodiscs.
- (2)
- (2)
- (2)
- (2)
- (2)
- (2)
- (2)
- (2)
- (2)
- (1)
Scaffold Protein Variants
The prevalently used nanodisc scaffold protein variant is MSP1D1. The protein comprises 10 a-helices similar to Apo A-I but with a modified N-terminus and increased stability of the assembled disc compared to the original MSP1. MSP1D1-deltaH5 corresponds to MSP1D1 except for a deletion of helix 5, thus resulting in a nanodisc complex with smaller diameter more suitable e.g. for NMR studies. MSP1E3D1 on the other hand has been extended by a duplication of three helices whereas MSP2N2 corresponds to a full length MSP1D1 fused via a short linker to a truncated MSP1D2 protein. These extended forms render larger diameter nanodiscs possible that are suitable for the incorporation of larger protein complexes.
MSP Nanodisc Assembly
Nanodiscs self-assemble from a mixture of the MSPs, phospholipids, and detergent upon removal of the detergent. The optimal ratio of the phospholipid to MSP is a critical parameter to assure complete self-assembly and homogenously sized nanodiscs. This ratio varies depending on the utilized MSP as well as the phospholipid. Furthermore, carefully controlling the molar ration between the target protein, the MSP, and lipids it is possible to assemble nanodiscs with different oligomeric state of the membrane protein (5).
MSP1D1 | MSP1E3D1 | Incubation at | |
---|---|---|---|
DPPC | 90:1 | 170:1 | 37°C |
DMPC | 80:1 | 150:1 | 25°C |
POPC | 65:1 | 130:1 | 4°C |
Purified membrane proteins are spontaneously reconstituted upon addition of MSPs and phospholipids and removal of the detergent. In case of membrane bound proteins membrane phospholipids form also the bilayer in the nanodisc surrounding the protein after assembly. The presence of the native phospholipids can positively affect protein activity and structure. Furthermore, the exposure of the solubilized protein to detergent is significantly shorter.
In order to reconstitute membrane proteins in a nanodiscs, the protein can be expressed in a cell-free system in the presence of an assembled nanodiscs (Fig.2 A). Alternatively, the purified protein can be solubilized using an appropriate detergent (Fig.2B). In a subsequent step, MSPs are and phospholipids are added to. Nanodiscs containing the membrane protein form spontaneously. A third option is to reconstitute the protein of interest in a nanodisc directly from membranes (Fig.2 C). Protein-nanodisccomplexes can be purified from those in the original membrane by affinity chromatography via the His-tag on the MSP. The exposure time for (C) is considerably shorter than for (B).
Phospholipids
The phospholipid bilayer inside the nanodisc can be composed of different phospholipids or phospholipid mixtures to best suit the protein of interest. The lipid selection depends heavily on the protein and can have significant influence on its activity. Furthermore, proteins from different species require specific phospholipids: e.g. a human membrane protein typically requires a different phospholipid environment than a bacterial membrane protein. It is therefore advisable to screen various different phospholipids.
Commonly used phospholipids are palmitoyl-oleoyl-phosphatidylcholine (POPC), dimyristoyl-glycero-phosphocholine (DMPC), or dimyristoyl-phosphatidyl-glcyol (DMPG) 1,2-dipalmitoyl-sn-glycero-3-phosphocholine (DPPC). There is however a plethora of phospholipids available to ideally accommodate the characteristics of your protein of interest.
Synthetic Nanodiscs
Unlike MSP Nanodiscs, synthetic Nanodiscs are formed using synthetic polymers, typically styrene-maleic acid co-polymers (SMAs) and Diisobutylene-maleic acid (DIBMA). Each polymer presents distinct advantages and limitations. For instance, DIBMA does not absorb light at 280 nm, making it particularly suitable for protein analysis involving spectroscopic techniques. The assembly process for synthetic Nanodisc proteins begins with intact cells, where the long polymer chains integrate into the cell membrane around the target MPs. This interaction detaches the MPs from the membrane, allowing them to dissolve in the polymer solution. The polymer-protein interaction stabilizes the MPs within the newly formed Nanodiscs. In summary, the use of synthetic polymers offers greater flexibility and control in stabilizing and analyzing MPs, enabling the production of stable and functional Nanodiscs for a wide range of biochemical and biophysical research applications.
Available Synthetic Nanodisc Variants
antibodies-online offers following Synthetic Nanodiscs. Unlike other MSP Nanodisc on the market, our Synthetic Nanodisc can be prepared directly from the cells. The polymers used during this process have a dual function. It dissolves the cell membranes, like the detergent, and uses cellular phospholipids to form Nanodisc around the membrane proteins. The target protein embedded Nanodiscs can then be purified.
- (3)
- (3)
- (3)
- (3)
- (2)
- (2)
- (2)
- (2)
- (2)
- (2)
Which Type of Nanodisc for Which Protein?
Altough both, MSP and Synthetic Nanodisc aim to achieve solubilization and stabilization of MPs there are distinct differences between them. They define the best use cases for each Nanodisc. Discover the table below to understand the differences and learn which nanodisc is suitable for which application.
MSP Nanodisc Protein | Synthetic Nanodisc Protein | |
---|---|---|
Stabilizer | MSP | Polymer |
Size | 7-17nm | Around 10nm |
Lipid composition | Artificial phospholipid environments | Native cell membrane lipids |
Protein sources | Proteins obtained from Cell-free reaction or detergent-solubilized method | Directly from the cells |
Involvement of detergent | May use detergent during protein preparation | No detergent |
MSP Nanodisc proteins have a uniform size, making them ideal for applications like Cryo-EM. In contrast, polymer-based Nanodiscs may require additional screening because the polymer diameters used in their formation can vary.
A significant challenge with MSP Nanodiscs is the interference caused by the MSP protein itself. The concentration of the target protein cannot be easily determined by measuring absorbance at 280 nm, and MSP can introduce background noise when used in assays alongside the target protein.
Additionally, when working with MSP Nanodiscs, detergents are used to dissolve the cell membrane, so it is essential to choose a detergent that does not disrupt the folding of the target protein. In contrast, Synthetic Nanodiscs do not require detergents, as the polymers used serve both as solubilizers and stabilizers. Learn more how Nanodisc are utilized in papers in different applications in the following paragraph.
Applications
Nanodiscs cater to any application in which membrane proteins in their native state in a model membrane system are mandatory. The following table gives examples where Nanodiscs have been used to study proteins along with the utilized application.
Application | Protein studied | Origin | Reference |
---|---|---|---|
Agonist/antagonist binding and radiolabel exchange in G proteins | beta andrenergic receptor 2 | human | (6) |
Surface plasmon resonance (SPR) | CD4 mutant | human | (7) |
Resonance Raman spectroscopy | cytochrome P450 | mammalian | (8) |
Time-resolved fluorescence spectroscopy | light harvesting complex II (LHCII) | spinach | (9) |
Matrix assisted laser desorption/ionization mass spectrometry (MALDI-MS) | various | various | (10,11) |
Non-covalent mass spectrometry (LILBID-ESI-MS) | KcsA LspA, EmrE, Hv1, proteorhodopsin, MraY | human bacteria | (12) |
Electron microscopy (EM) | light harvesting complex II (LHCII) | spinach | (13) |
Cryo electron microscopy (EM) | cySecYEG complex, TcdA1 toxin complex | bacteria | (14, 15) |
Mouse vaccination | hemagglutinin (HA) | influenza virus | (16) |
Nuclear magnetic resonance (NMR) | OmpX CD4 mutant | human bacteria | (17, 18) |
NMR analysis of lipid-interacting, soluble proteins | phospho-inositol binding proteins | human | (19) |
Transfer into bicelles for NMR | lipoprotein signal peptidase II (LspA) | human | (20) |
Protein phosphorylation / activation studies | epidermal growth factor receptor (EGFR) | human | (21) |
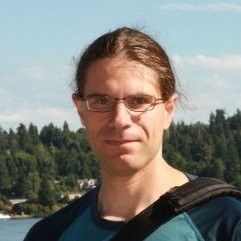
Goal-oriented, time line driven scientist, proficiently trained in different academic institutions in Germany, France and the USA. Experienced in the life sciences e-commerce environment with a focus on product development and customer relation management.
Go to author page